【溫馨提示】壓縮包內(nèi)含CAD圖有下方大圖片預覽,下拉即可直觀呈現(xiàn)眼前查看、盡收眼底縱觀。打包內(nèi)容里dwg后綴的文件為CAD圖,可編輯,無水印,高清圖,壓縮包內(nèi)文檔可直接點開預覽,需要原稿請自助充值下載,所見才能所得,請見壓縮包內(nèi)的文件及下方預覽,請細心查看有疑問可以咨詢QQ:11970985或197216396
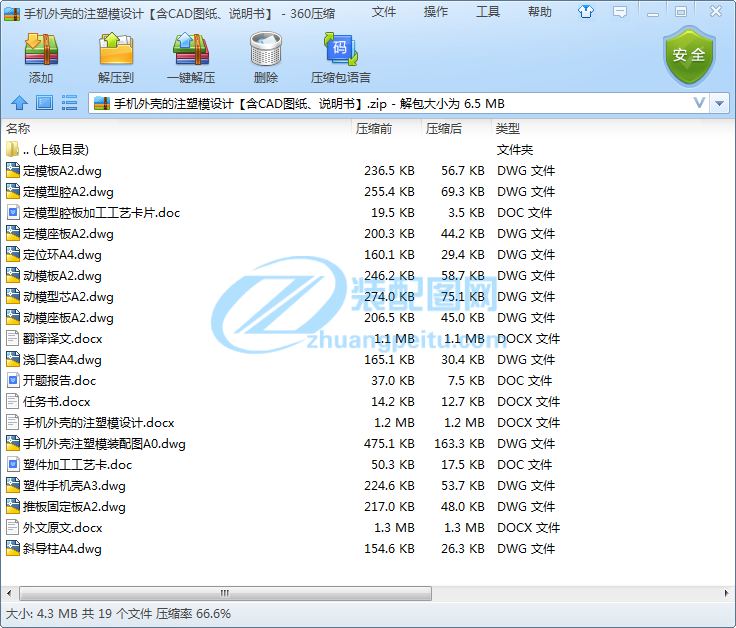
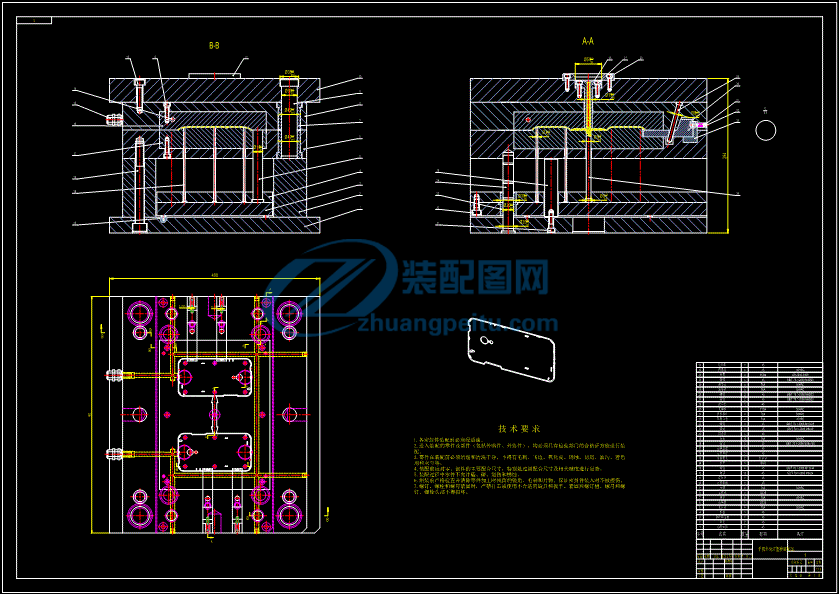
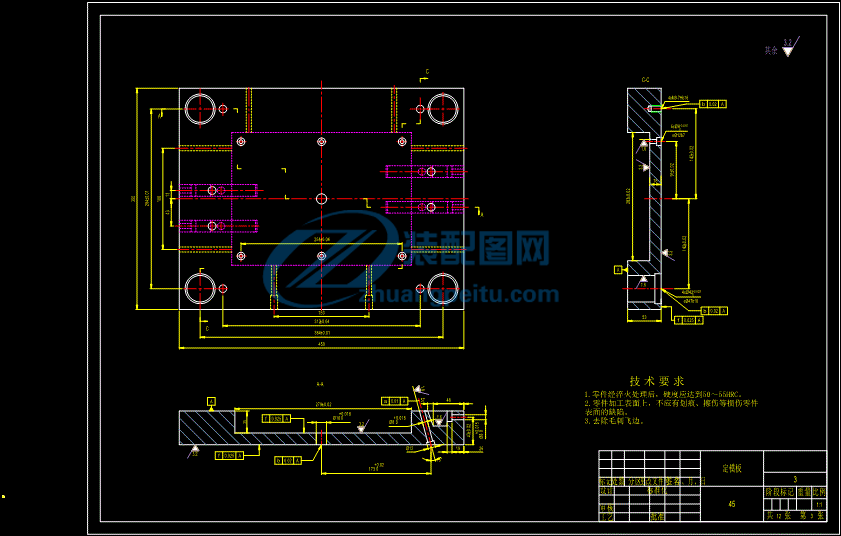
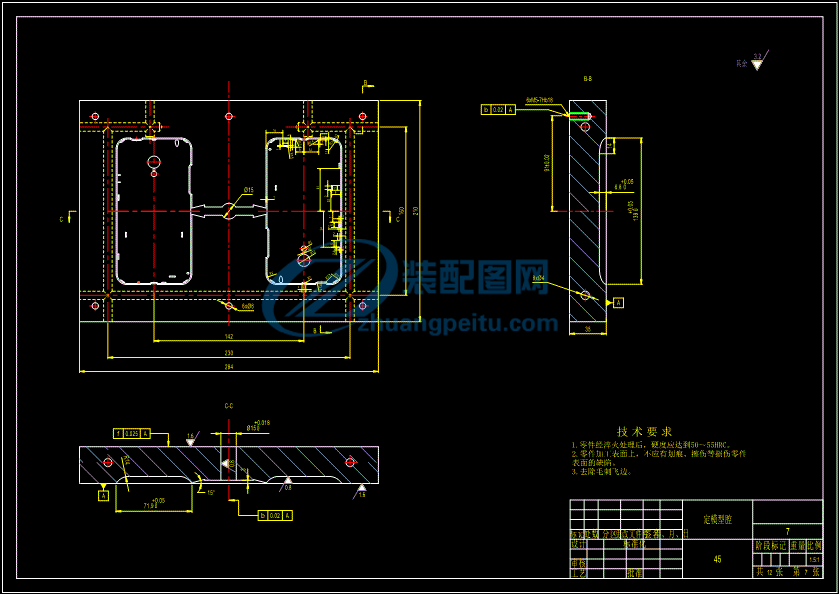
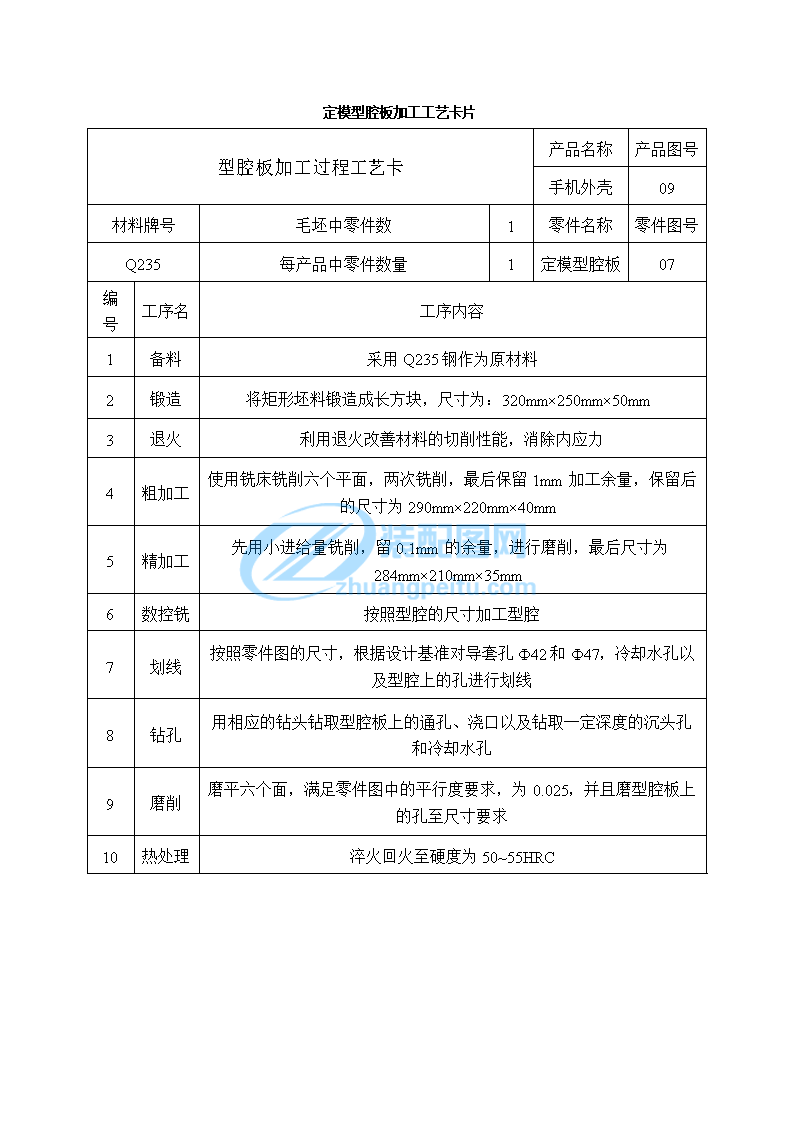
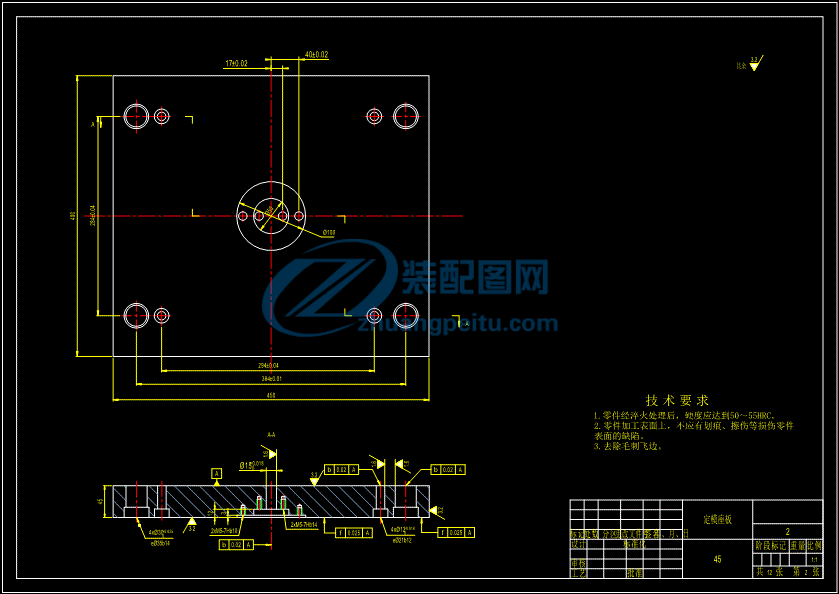
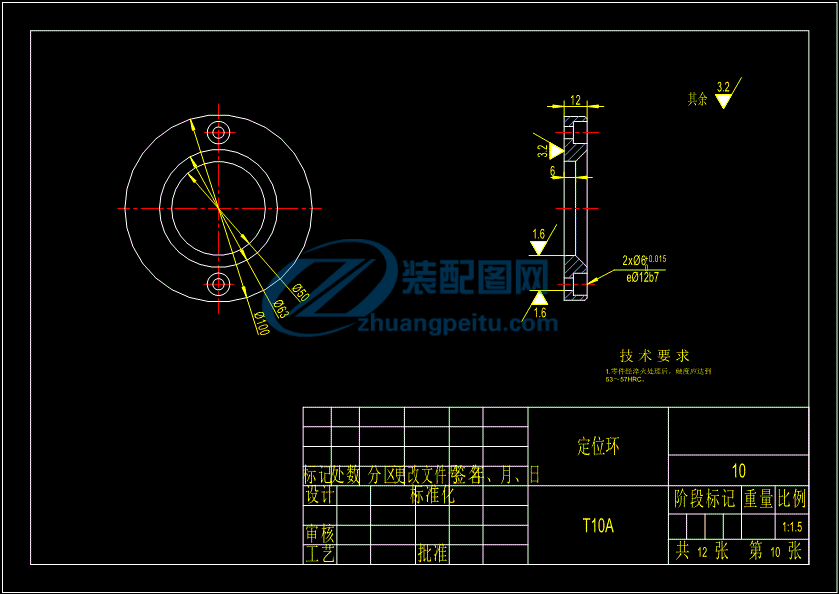
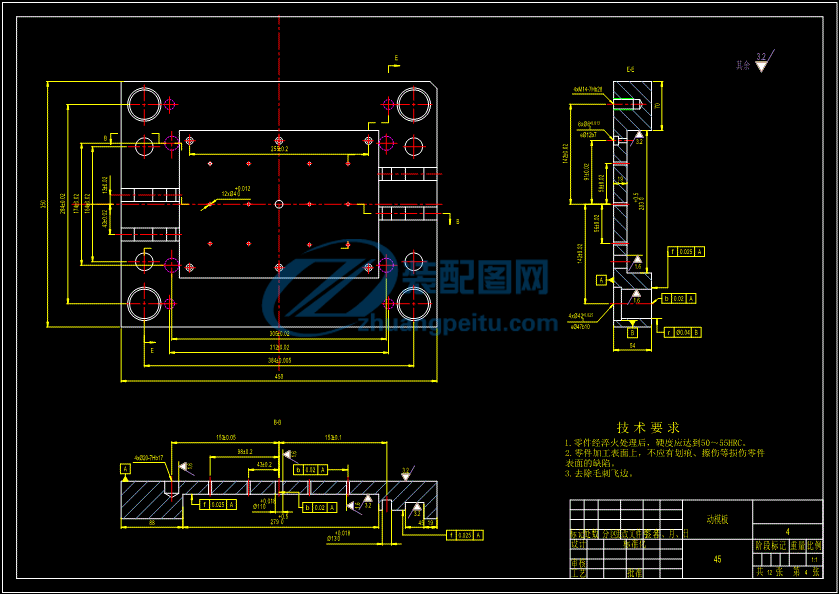
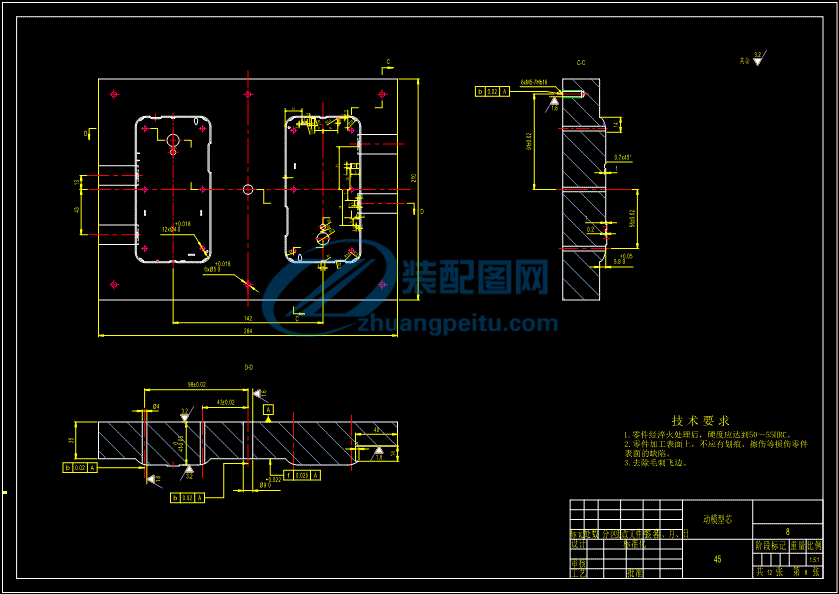
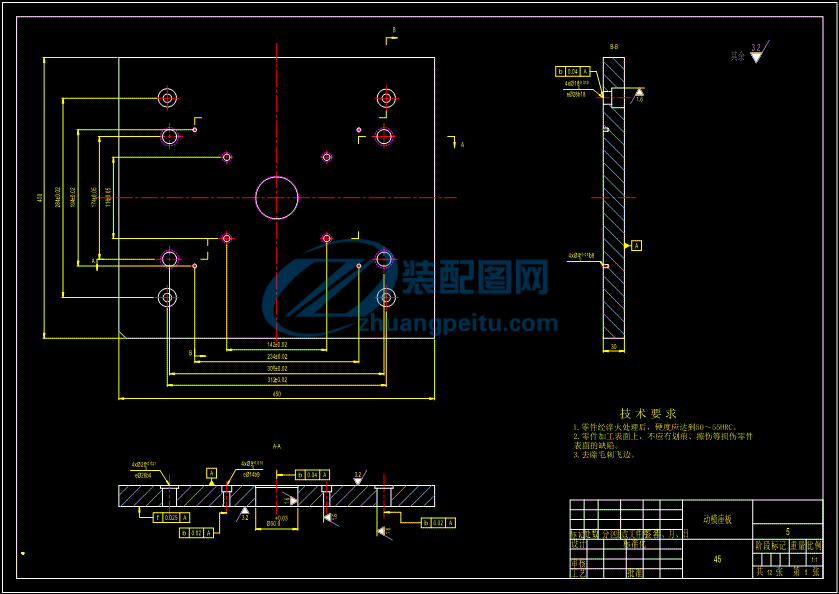
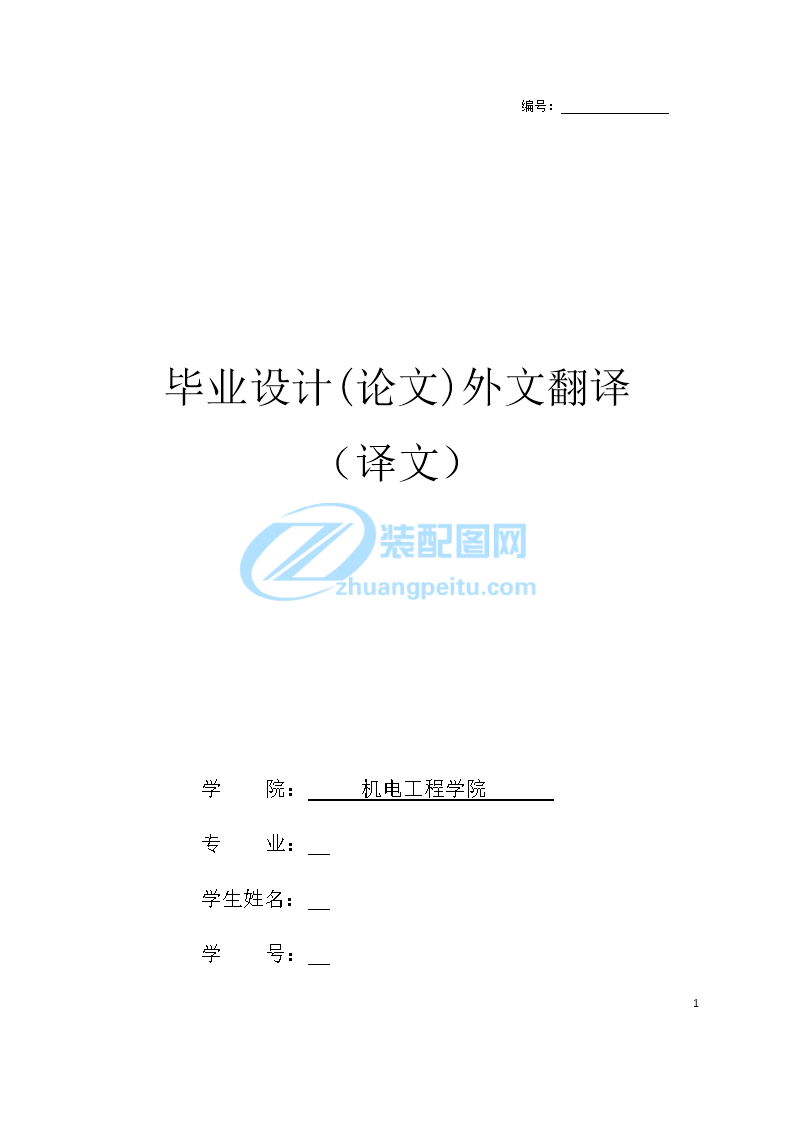
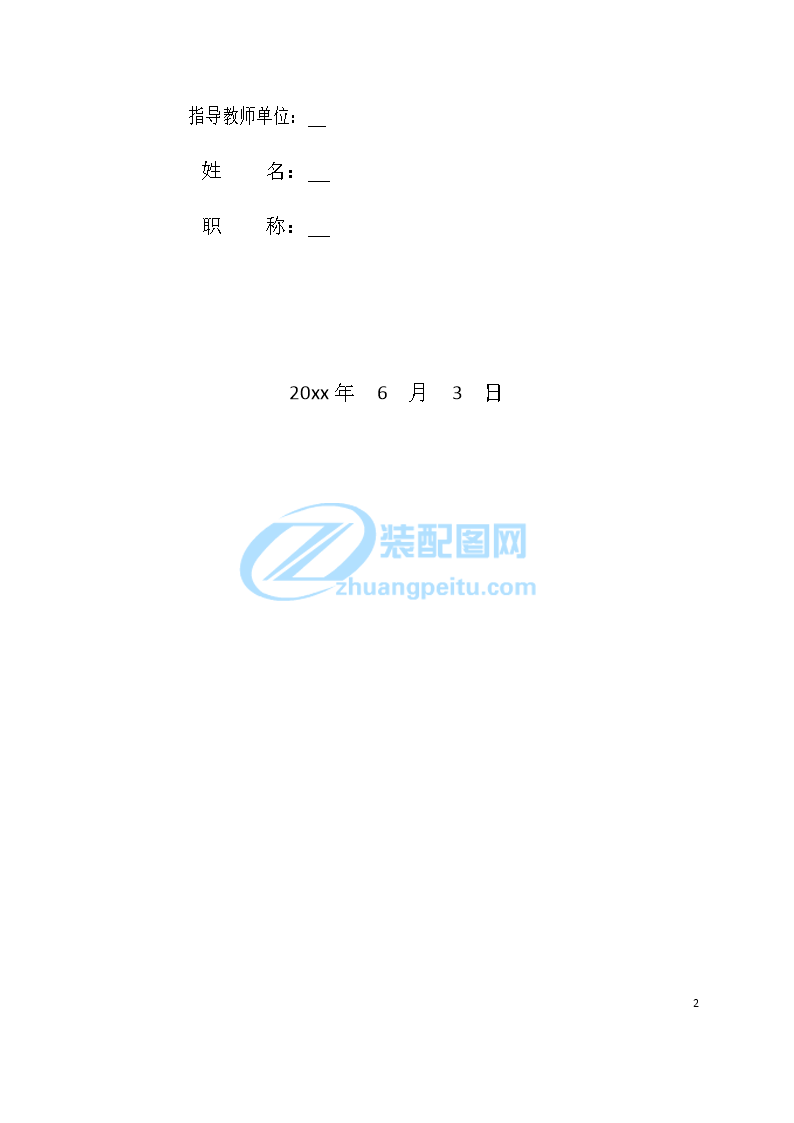
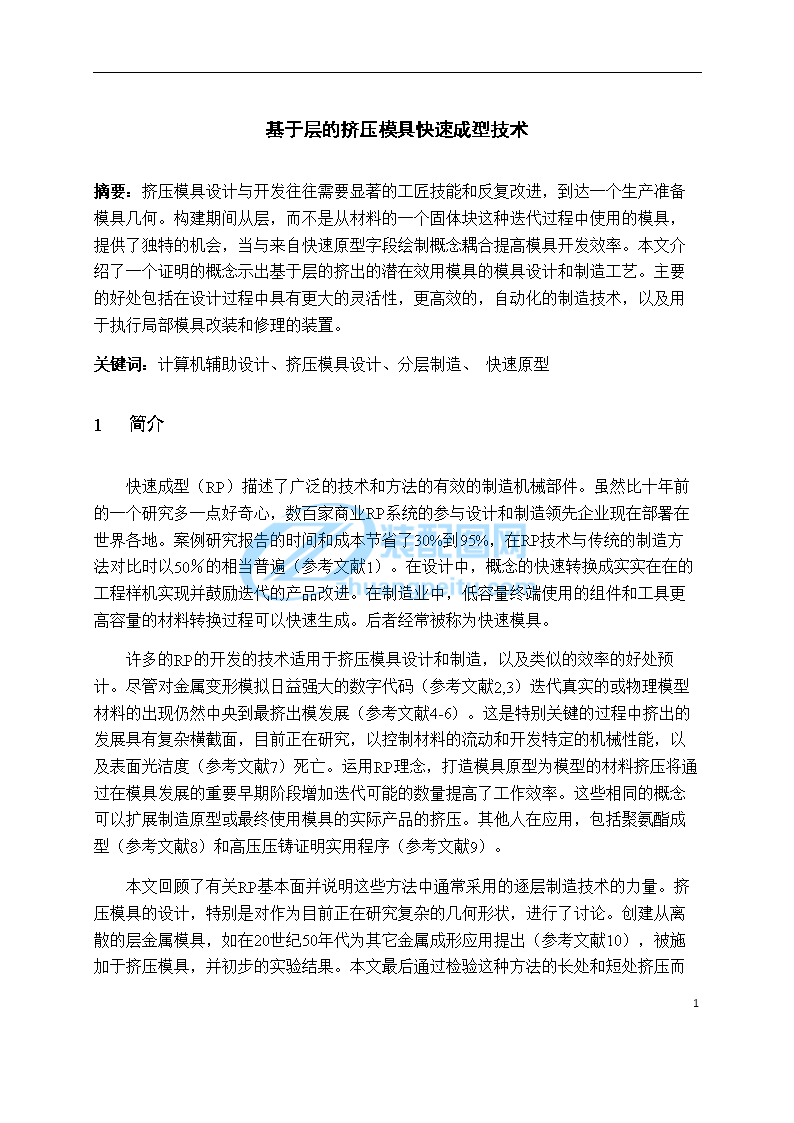
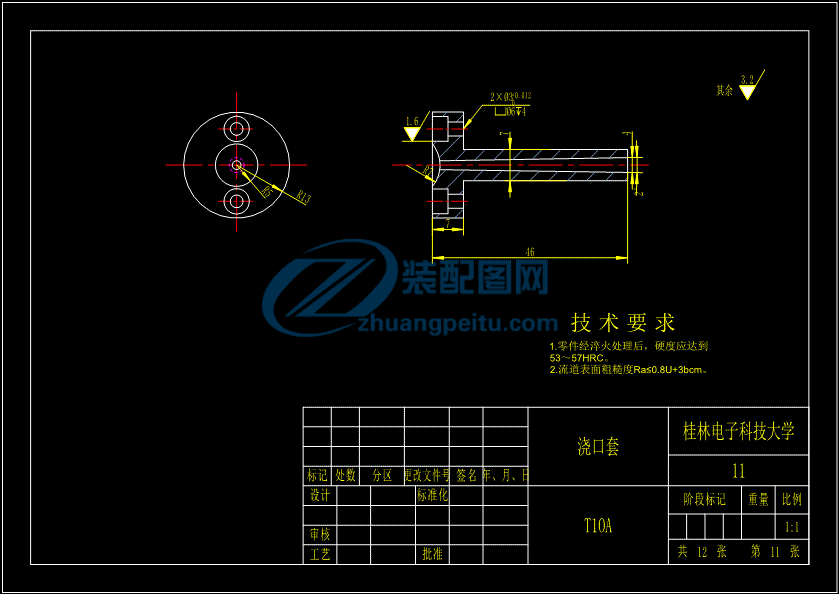
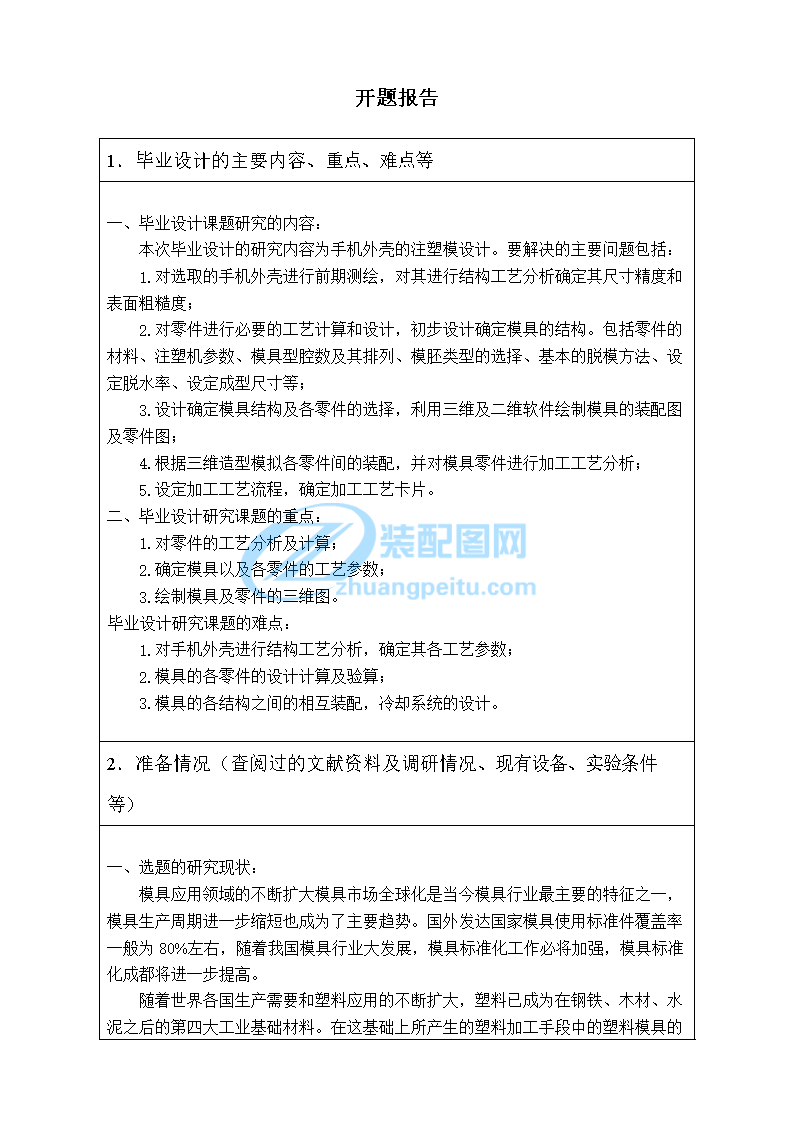
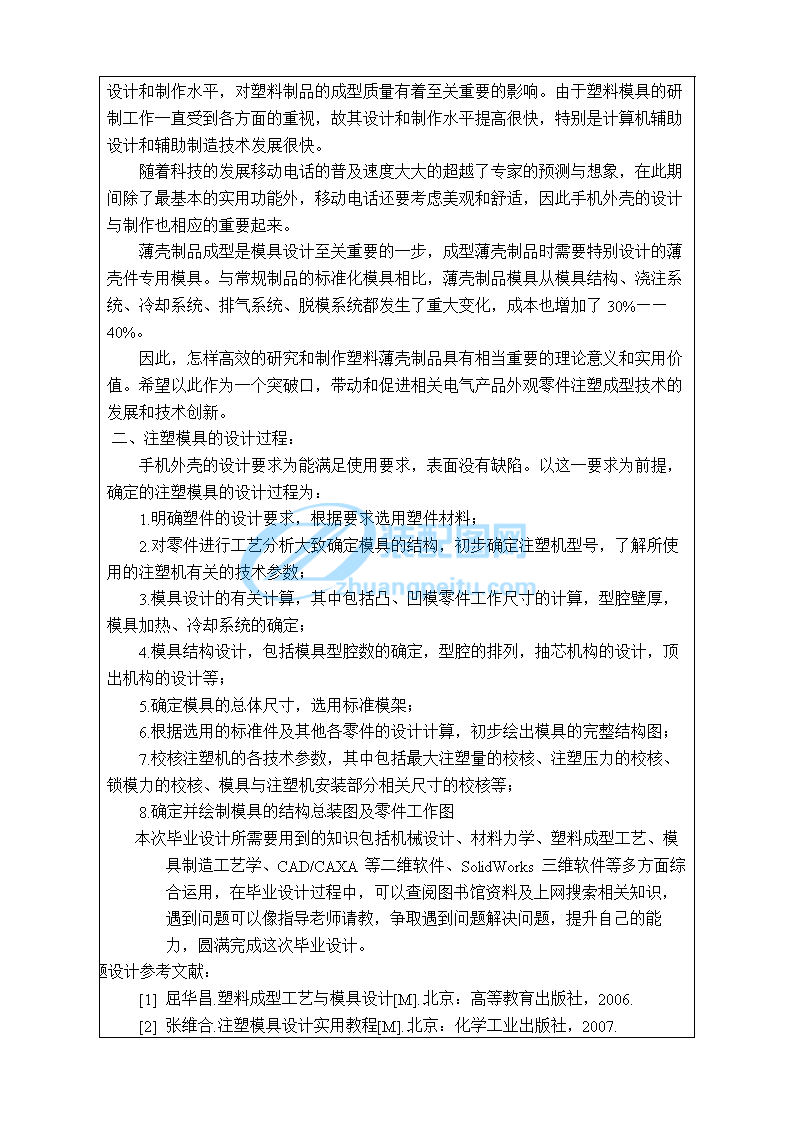
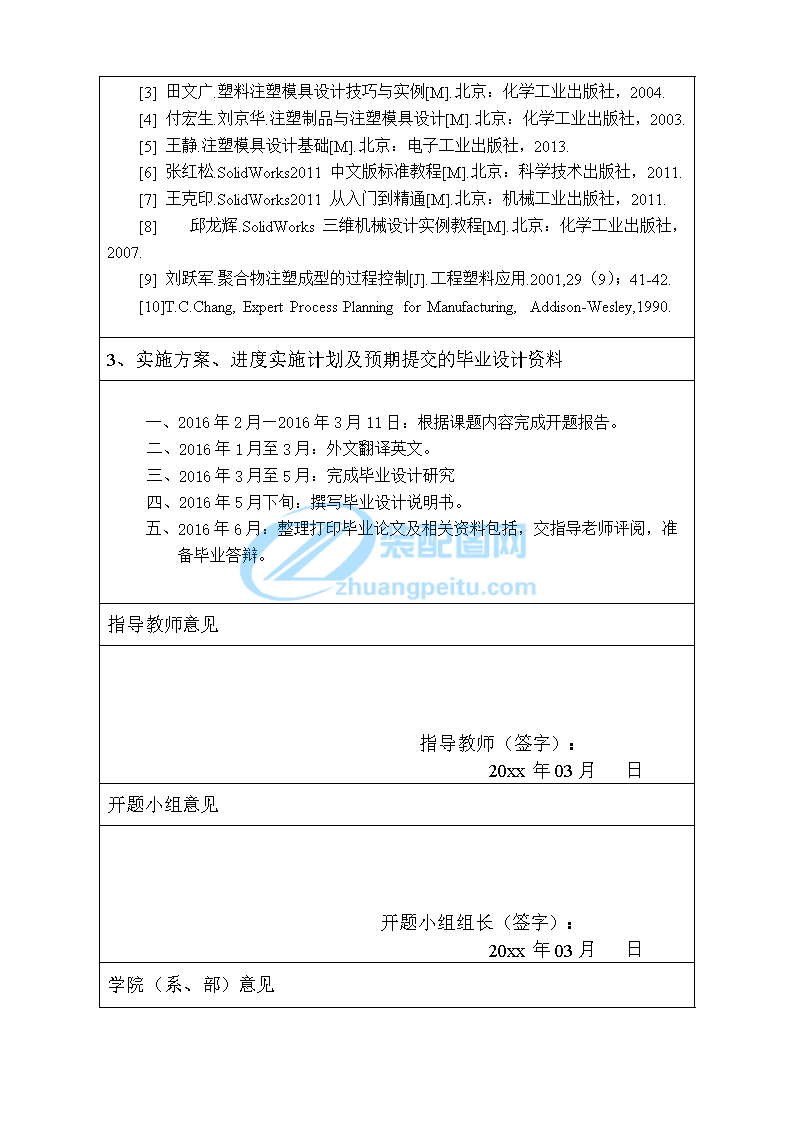
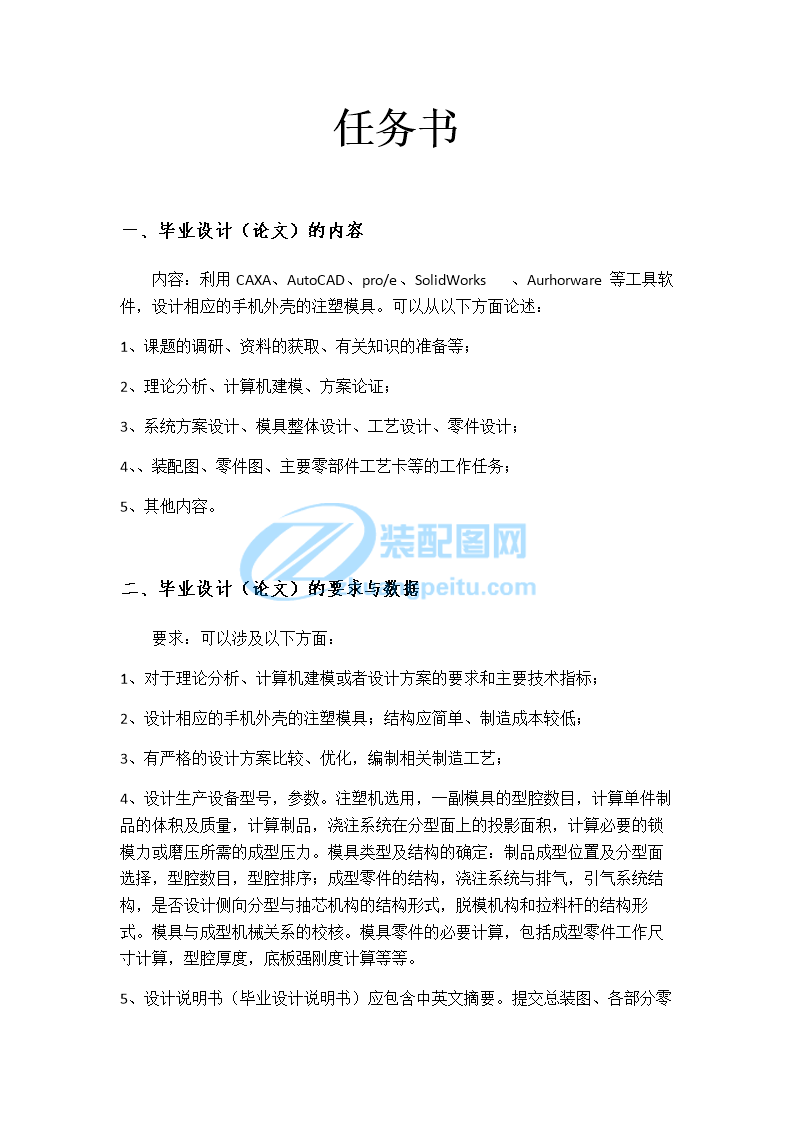
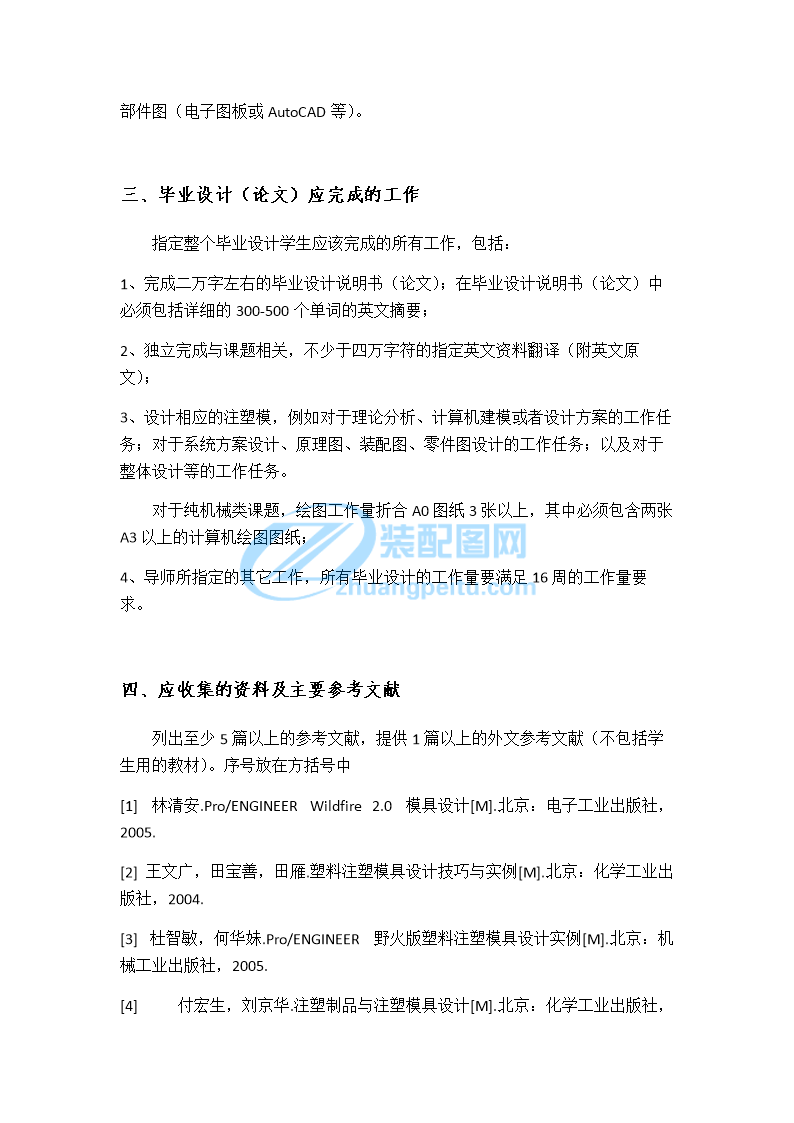
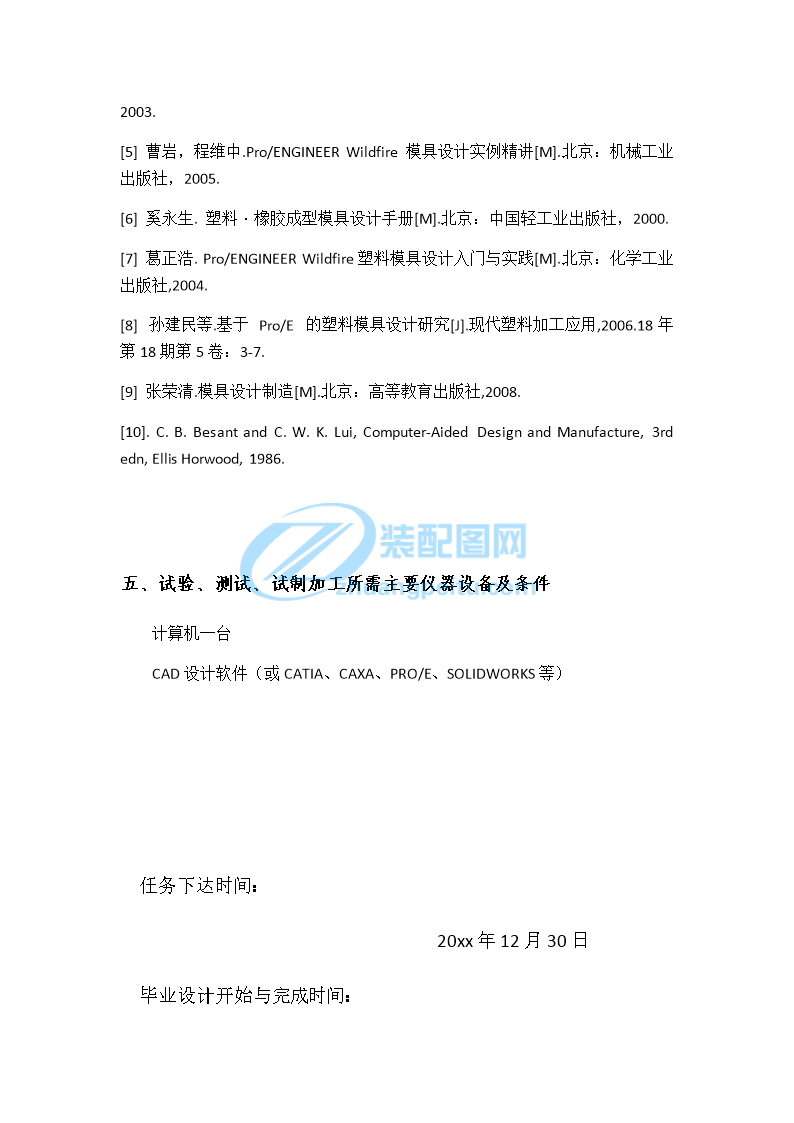
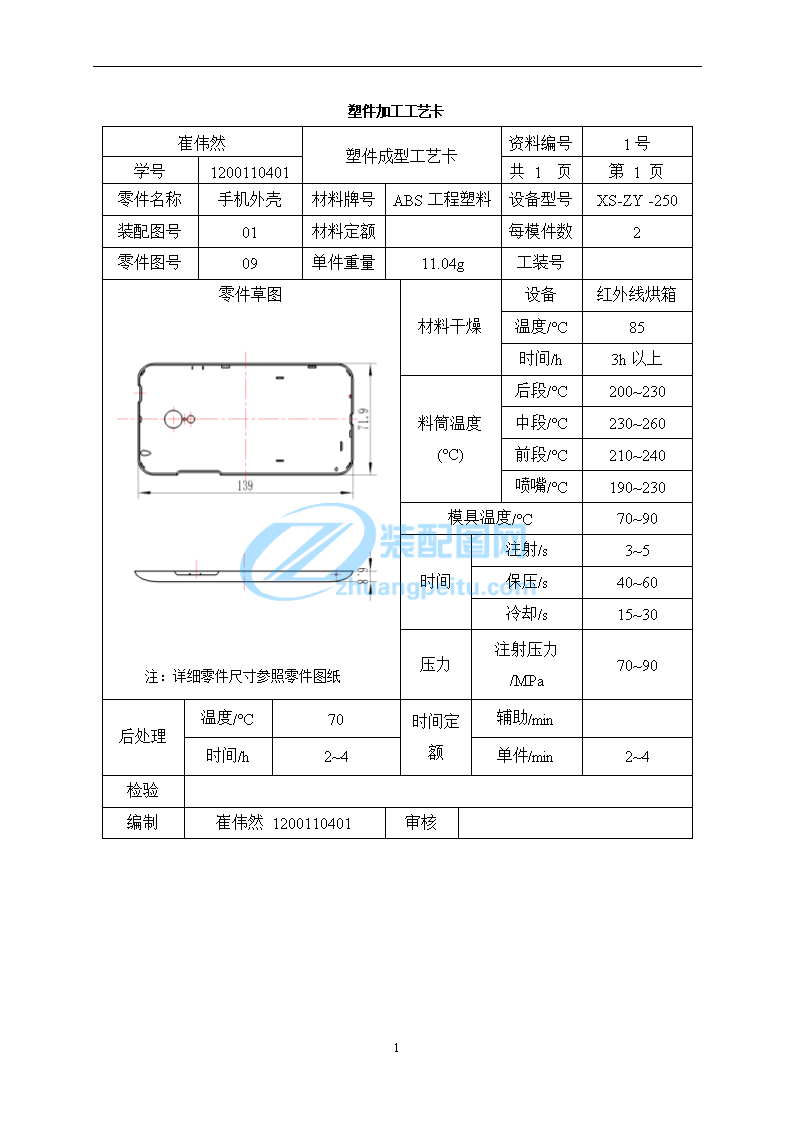
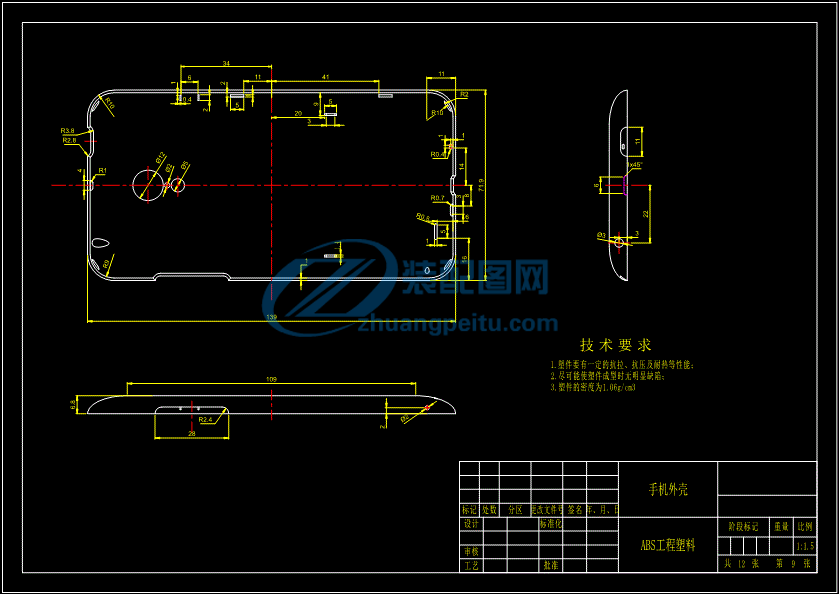
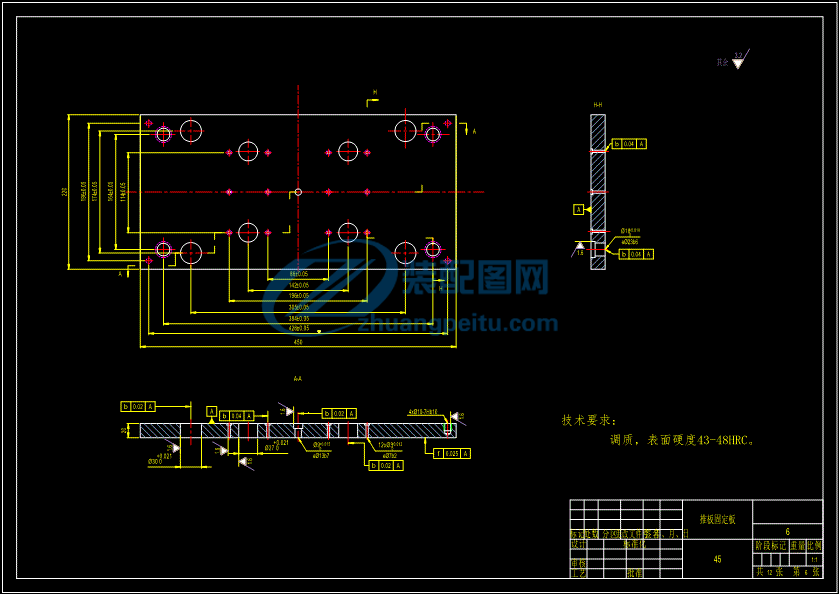
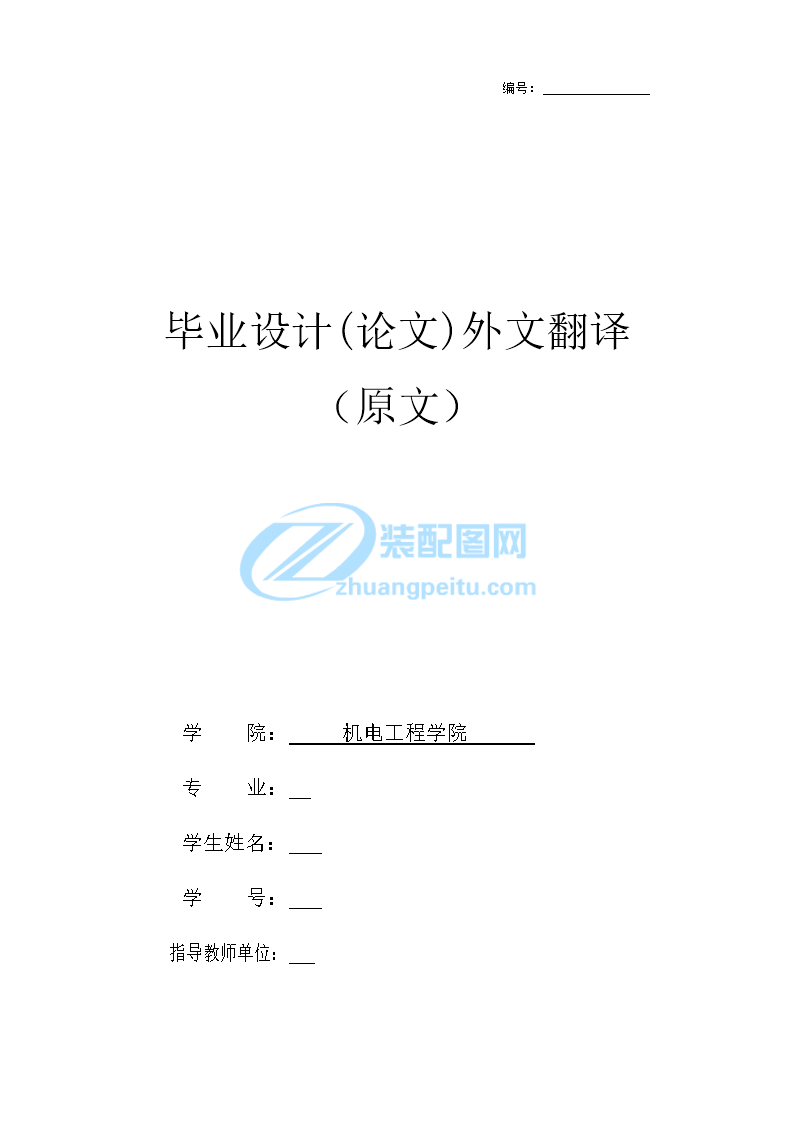
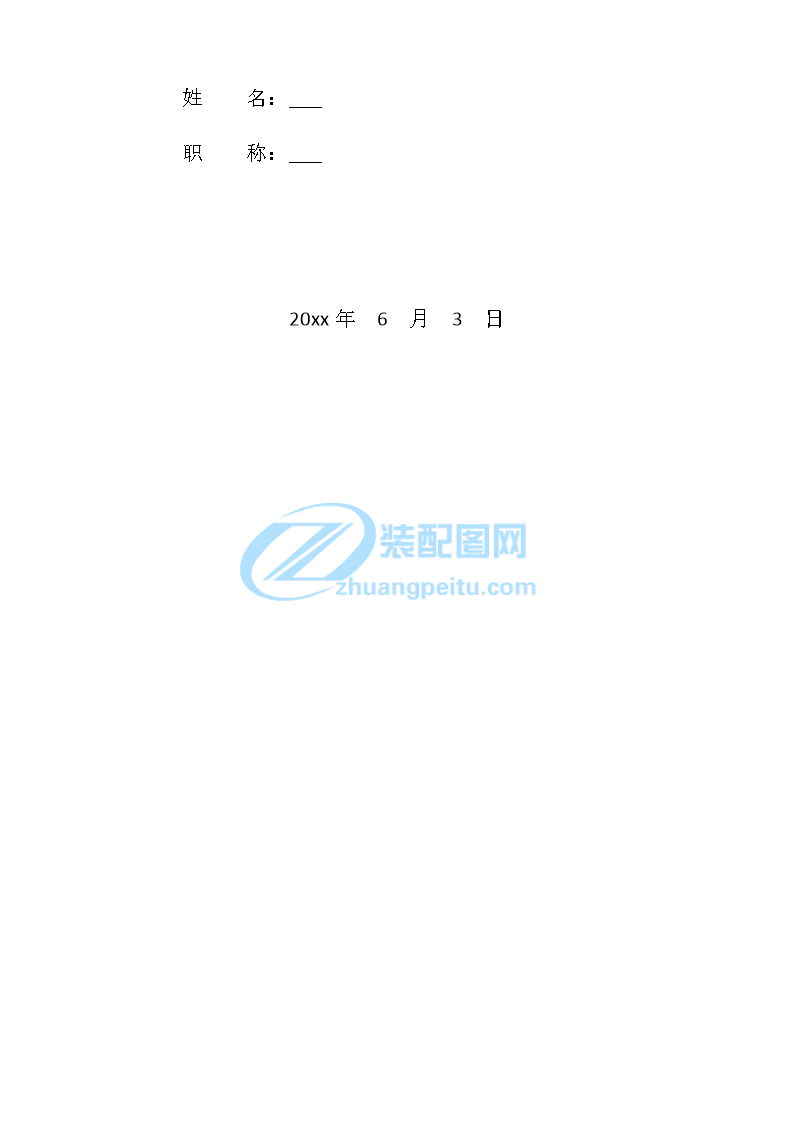
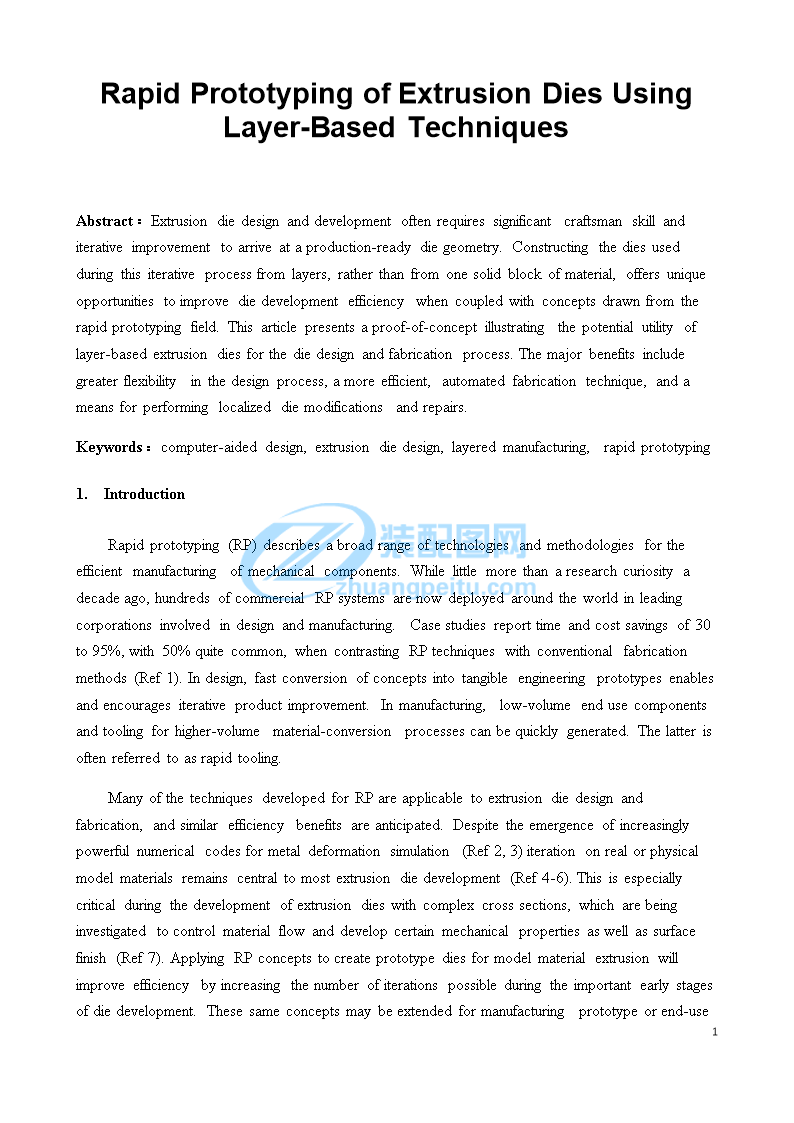
任務書
一、畢業(yè)設計(論文)的內(nèi)容
內(nèi)容:利用CAXA、AutoCAD、pro/e、SolidWorks 、Aurhorware等工具軟件,設計相應的手機外殼的注塑模具??梢詮囊韵路矫嬲撌觯?
1、課題的調(diào)研、資料的獲取、有關(guān)知識的準備等;
2、理論分析、計算機建模、方案論證;
3、系統(tǒng)方案設計、模具整體設計、工藝設計、零件設計;
4、、裝配圖、零件圖、主要零部件工藝卡等的工作任務;
5、其他內(nèi)容。
二、畢業(yè)設計(論文)的要求與數(shù)據(jù)
要求:可以涉及以下方面:
1、對于理論分析、計算機建?;蛘咴O計方案的要求和主要技術(shù)指標;
2、設計相應的手機外殼的注塑模具;結(jié)構(gòu)應簡單、制造成本較低;
3、有嚴格的設計方案比較、優(yōu)化,編制相關(guān)制造工藝;
4、設計生產(chǎn)設備型號,參數(shù)。注塑機選用,一副模具的型腔數(shù)目,計算單件制品的體積及質(zhì)量,計算制品,澆注系統(tǒng)在分型面上的投影面積,計算必要的鎖模力或磨壓所需的成型壓力。模具類型及結(jié)構(gòu)的確定:制品成型位置及分型面選擇,型腔數(shù)目,型腔排序;成型零件的結(jié)構(gòu),澆注系統(tǒng)與排氣,引氣系統(tǒng)結(jié)構(gòu),是否設計側(cè)向分型與抽芯機構(gòu)的結(jié)構(gòu)形式,脫模機構(gòu)和拉料桿的結(jié)構(gòu)形式。模具與成型機械關(guān)系的校核。模具零件的必要計算,包括成型零件工作尺寸計算,型腔厚度,底板強剛度計算等等。
5、設計說明書(畢業(yè)設計說明書)應包含中英文摘要。提交總裝圖、各部分零部件圖(電子圖板或AutoCAD等)。
三、畢業(yè)設計(論文)應完成的工作
指定整個畢業(yè)設計學生應該完成的所有工作,包括:
1、完成二萬字左右的畢業(yè)設計說明書(論文);在畢業(yè)設計說明書(論文)中必須包括詳細的300-500個單詞的英文摘要;
2、獨立完成與課題相關(guān),不少于四萬字符的指定英文資料翻譯(附英文原文);
3、設計相應的注塑模,例如對于理論分析、計算機建?;蛘咴O計方案的工作任務;對于系統(tǒng)方案設計、原理圖、裝配圖、零件圖設計的工作任務;以及對于整體設計等的工作任務。
對于純機械類課題,繪圖工作量折合A0圖紙3張以上,其中必須包含兩張A3以上的計算機繪圖圖紙;
4、導師所指定的其它工作,所有畢業(yè)設計的工作量要滿足16周的工作量要求。
四、應收集的資料及主要參考文獻
列出至少5篇以上的參考文獻,提供1篇以上的外文參考文獻(不包括學生用的教材)。序號放在方括號中
[1] 林清安.Pro/ENGINEER Wildfire 2.0 模具設計[M].北京:電子工業(yè)出版社,2005.
[2] 王文廣,田寶善,田雁.塑料注塑模具設計技巧與實例[M].北京:化學工業(yè)出版社,2004.
[3] 杜智敏,何華妹.Pro/ENGINEER 野火版塑料注塑模具設計實例[M].北京:機械工業(yè)出版社,2005.
[4] 付宏生,劉京華.注塑制品與注塑模具設計[M].北京:化學工業(yè)出版社,2003.
[5] 曹巖,程維中.Pro/ENGINEER Wildfire 模具設計實例精講[M].北京:機械工業(yè)出版社,2005.
[6] 奚永生. 塑料·橡膠成型模具設計手冊[M].北京:中國輕工業(yè)出版社,2000.
[7] 葛正浩. Pro/ENGINEER Wildfire塑料模具設計入門與實踐[M].北京:化學工業(yè)出版社,2004.
[8] 孫建民等.基于 Pro/E 的塑料模具設計研究[J].現(xiàn)代塑料加工應用,2006.18年第18期第5卷:3-7.
[9] 張榮清.模具設計制造[M].北京:高等教育出版社,2008.
[10]. C. B. Besant and C. W. K. Lui, Computer-Aided Design and Manufacture, 3rd edn, Ellis Horwood, 1986.
五、試驗、測試、試制加工所需主要儀器設備及條件
計算機一臺
CAD設計軟件(或CATIA、CAXA、PRO/E、SOLIDWORKS等)
任務下達時間:
20xx年12月30日
畢業(yè)設計開始與完成時間:
20xx年12月27日至 20xx年05 月26日
組織實施單位:
教研室主任意見:
簽字: 20xx年1月8 日
院領(lǐng)導小組意見:
簽字: 20xx 年1 月16日
塑件加工工藝卡
崔偉然
塑件成型工藝卡
資料編號
1號
學號
1200110401
共 1 頁
第 1 頁
零件名稱
手機外殼
材料牌號
ABS工程塑料
設備型號
XS-ZY -250
裝配圖號
01
材料定額
每模件數(shù)
2
零件圖號
09
單件重量
11.04g
工裝號
零件草圖
注:詳細零件尺寸參照零件圖紙
材料干燥
設備
紅外線烘箱
溫度/°C
85
時間/h
3h以上
料筒溫度(°C)
后段/°C
200~230
中段/°C
230~260
前段/°C
210~240
噴嘴/°C
190~230
模具溫度/°C
70~90
時間
注射/s
3~5
保壓/s
40~60
冷卻/s
15~30
壓力
注射壓力/MPa
70~90
后處理
溫度/°C
70
時間定額
輔助/min
時間/h
2~4
單件/min
2~4
檢驗
編制
崔偉然 1200110401
審核
2
編號:
畢業(yè)設計(論文)外文翻譯
(原文)
學 院: 機電工程學院
專 業(yè):
學生姓名:
學 號:
指導教師單位:
姓 名:
職 稱:
20xx年 6 月 3 日
Rapid Prototyping of Extrusion Dies Using Layer-Based Techniques
Abstract:Extrusion die design and development often requires significant craftsman skill and iterative improvement to arrive at a production-ready die geometry. Constructing the dies used during this iterative process from layers, rather than from one solid block of material, offers unique opportunities to improve die development efficiency when coupled with concepts drawn from the rapid prototyping field. This article presents a proof-of-concept illustrating the potential utility of layer-based extrusion dies for the die design and fabrication process. The major benefits include greater flexibility in the design process, a more efficient, automated fabrication technique, and a means for performing localized die modifications and repairs.
Keywords:computer-aided design, extrusion die design, layered manufacturing, rapid prototyping
1. Introduction
Rapid prototyping (RP) describes a broad range of technologies and methodologies for the efficient manufacturing of mechanical components. While little more than a research curiosity a decade ago, hundreds of commercial RP systems are now deployed around the world in leading corporations involved in design and manufacturing. Case studies report time and cost savings of 30 to 95%, with 50% quite common, when contrasting RP techniques with conventional fabrication methods (Ref 1). In design, fast conversion of concepts into tangible engineering prototypes enables and encourages iterative product improvement. In manufacturing, low-volume end use components and tooling for higher-volume material-conversion processes can be quickly generated. The latter is often referred to as rapid tooling.
Many of the techniques developed for RP are applicable to extrusion die design and fabrication, and similar efficiency benefits are anticipated. Despite the emergence of increasingly powerful numerical codes for metal deformation simulation (Ref 2, 3) iteration on real or physical model materials remains central to most extrusion die development (Ref 4-6). This is especially critical during the development of extrusion dies with complex cross sections, which are being investigated to control material flow and develop certain mechanical properties as well as surface finish (Ref 7). Applying RP concepts to create prototype dies for model material extrusion will improve efficiency by increasing the number of iterations possible during the important early stages of die development. These same concepts may be extended for manufacturing prototype or end-use dies for real product extrusion. Others have demonstrated utility in applications including polyurethane molding (Ref 8) and pressure die-casting (Ref 9).
This article reviews the relevant RP fundamentals and illustrates the power of the layerwise manufacturing technique commonly applied by these processes. Extrusion die design, particularly for complex geometries as are currently being investigated, is discussed. Creating dies from discrete layers, as proposed in the 1950s for other metalforming applications (Ref 10), is applied for extrusion dies, and preliminary experimental results are presented. This article concludes by examining strengths and weaknesses of this approach for extrusion while outlining potential applications. W.Z. Misiolek, Institute for Metal Forming, Lehigh University, Bethlehem, PA 18015, USA; and K.T. Winther, A.E. Prats, and S.J. Rock, Rensselaer Polytechnic Institute, Troy, NY 12180, USA.
2. Rapid Prototyping
Rapid prototyping is defined as the rapid production of prototype models (Ref 11). Many commercial RP processes and industrial success stories have emerged in the last decade. Rapid prototyping represents both a methodology and group of technologies that have proven particularly effective at speeding the conversion of design information into physical reality. In general, this is achieved by standardizing the information required by manufacturing processes while automating the manner in which this defining information is processed. This automation is possible, in part, due to the development of a group of manufacturing processes with simplified information requirements. These are known synonymously as solid free-form fabrication (SFF) or solid freeform manufacturing (SFM) processes.
2.1 Solid Freeform Fabrication/Solid Freeform Manufacturing
Solid freeform fabrication or SFM processes have received substantial attention and are responsible for much of the recent growth in the application of RP. The key feature of any process classified as an SFF process is that it requires no part-specific tooling or human intervention to realize components (Ref 12). The attempts to satisfy this goal have stimulated the development of many processes with greatly simplified informational requirements, and this facilitates a more automatic push-button method for component manufacture. Many SFF processes, for example, make use of layered manufacturing techniques (LMTs) which ultimately require only planar information, rather than three-dimensional information, to control process hardware.
2.2 Layered Manufacturing Techniques
Solid components are created using LMTs by additively building up planar material layers corresponding to component cross sections. Layer-based techniques were proposed in the 1950s for making hydroforming tooling (Ref 10), and they have been studied more recently for other tooling applications (Ref 8, 9, 13, 14). Layer-based techniques have also proven quite useful in the context of RP, specifically given the tool and operator independence goals of SFF.
Interest in using LMTs for prototype components began with nonstructural materials such as paper, wax, and plastic; however, metal, ceramic, and composite components are now being produced using these techniques. Most RP processes based on LMTs utilize raw material layers on the order of hundreds of microns (several thousandths of an inch) to construct components. These techniques benefit from being able to create components of nearly unrestricted shape. Most processes are self-fixturing, and by constructing parts from thin layers, tool accessibility limitations common during machining are not experienced. Special case fixturing and tool path planning are rarely a consideration or obstacle to productivity when using these processes. Additionally, using such thin layers allows each contour of the layers comprising the part to be cut without regard to variability in the slope of the layer around its periphery. This further simplifies the information necessary to drive the layer-based process. Unfortunately, fabricating components using very thin layers is not without drawbacks. The number of layers required to form a typical part is large and the time required to process each layer can be significant. Approximations using edges perpendicular to each layer are common and produce spatial aliasing, also known as stair stepping, which is undesirable in certain situations.
Fig.1 Layered manufacturing techniques (Ref 16)
Fig. 2 Extrusion die cross sections. (a) Conventional. (b) Con-toured edge layered die. (c) Perpendicular edge layered die
Some applications using much thicker layers have been re-ported. Ford Motor Company used LMTs to produce a prototype engine block using numerically controlled (NC) machined 1 in. thick steel layers bonded using a special diffusion bonding operation (Ref 15). This eliminates many tool accessibility difficulties of machining components with complex internal passages and deep pockets, while retaining many of the benefits associated with time-tested machining such as the ability to machine a wide variety of materials, achieve high tolerances, and control surface finish. Many trade-offs between material thickness, manufacturing process, and target material must certainly be considered when selecting an appropriate LMT process
2.3 Driving Information
The power of LMTs and RP in general stems from the ability to quickly transform a geometrically complex three-dimensional design into the low-level control instructions necessary to guide an information-driven manufacturing process. Computer-aided design (CAD) solid models, which are computer-sensible representations of three-dimensional objects, are generally required to drive RP systems. Figure 1 illustrates how a CAD solid model is intersected to create slices corresponding to various layers comprising a final component. Note that while this example shape is quite simple for illustrative purposes, solid models representing more complex three-dimensional objects can be processed equally well using this technique. Increased geometric complexity will make the power of solid modeling fully evident.
The key requirement that has prevented some from enjoying the benefits of LMT is that three-dimensional solid models, rather than two-dimensional CAD or manual drawings, are required to efficiently drive these processes. However, improvements in desktop computing power, combined with the introduction of many new solid modeling packages, have today made three-dimensional CAD solid modeling capability accessible to even the smallest firms.
3. Layered Extrusion Dies
Constructing extrusion dies using discrete material layers offers many benefits similar to those realized for other applications of LMT (Ref 9). While envisioned principally as a tool to expedite the design and iterative refinement of extrusion dies, the proposed use of layered extrusion dies may also be applicable in production situations. This is an especially attractive approach for short production runs, where building a die using traditional methods would be economically prohibitive.
3.1 Die Development
Despite advancements in the die design and fabrication process, highly complex tooling is usually not perfected in one design iteration. It is quite often necessary to go through several trial and error iterations to introduce needed corrections into the die geometry. A major limitation of this approach is that these corrections are often performed manually and the original design is very seldom updated. Consequently, these modifications and the associated process knowledge are not easily captured and incorporated into future designs, and the general state of practice does not advance. This situation is exacerbated, and turnaround times can be lengthened substantially, when the die is manufactured outside of the extrusion plant. The LMT approach allows us to capture the necessary changes and easily incorporate them in all new designs.
3.2 Die Styles
Figure 2 contrasts a typical solid extrusion die and two forms of layered extrusion dies. The conventional die cross section shown in Fig. 2(a) illustrates a very smooth interior die surface, fabricated from a single piece of wrought stock. The layered extrusion die termed a contoured edge layered die shown in Fig. 2(b) offers a close approximation to this smooth, solid die. The smooth contour is approximated here by layers with straight edges around their periphery.
The layered extrusion die termed a perpendicular edge layered die offers an even simpler alternative. As shown in Fig. 2(c), this type of die approximates a smooth die contour using discrete layers with edges perpendicular to each layer about the periphery of each contour. Although this provides a poorer approximation to a smooth contour, the approximation offered by this die style is generally sufficient for the target application of extrusion. Localized dead metal zones will form in the corners created by the discrete die layers and serve to effectively smooth the die surface. Regardless of the type of approximation used, layered extrusion dies offer an attractive alternative to dies fabricated from one solid piece of material.
3.3 Die Fabrication
Contoured edge layered dies and perpendicular edge layered dies can be machined from planar stock. Both die styles can be cut using a three-axis NC machining center; however, the former is more efficiently cut using a five-axis mill to avoid requiring multiple fine passes to create sloped edges. Layer edges are best machined using the sides of an end mill. Figures 2(b) and (c) illustrate dies made from uniformly thick material layers, and this facilitates process standardization—in terms of stock material inventory, handling, fixturing, and tool path planning. However, it is possible to create a die using layers of varying thickness. In either case, a minimum layer thickness will be required for machining without complicated part fixturing.
Both die styles require planar contour information to define their shape. Perpendicular edge layered dies only require a single contour corresponding to the shape of each constituent layer; however, two contours are required to define the shape of each contoured edge layered die. Interpolation between these two contours can be used to determine the cut angle as a function of location about each pair of contours. If these dies are to be constructed of n layers, then either n or n + 1 contours, respectively, are required to fully define all die layers since mating faces of the contoured edge layered die will have identical contours. Contours representing a die design can be manually created, but additional advantages of layered dies will be realized when a CAD solid model is intersected by slice planes and the resulting slices used to automatically guide die layer machining. This is illustrated by Fig. 3.
Computer-aided design solid models are routinely transferred to RP systems using a polygonal approximate representation. Known as STL, this approximation can also be used in this application, and previous capability has been developed to slice these files (Ref 17). Slice contours must be offset to account for the cutter radius, and instructions that guide the NC milling machine, known as “NC code,” must be generated.
Provisions must be made to ensure proper alignment between successive die layers. A number of options such as interlocking features, keyways, or alignment pins can be used. Alignment pins provide an easy alternative since holes to accommodate each pin can be machined in each die layer while it is fixtured for edge profile cutting. This will lead to a high degree of alignment accuracy while being reasonably automated. A simple scheme for layer assemblage and alignment also will facilitate die modifications and maintenance.
3.4 Die Modifications
By constructing extrusion dies from discrete layers, localized die geometry changes can be made without having to manufacture an entirely new die. Figure 4 shows die contour half cross sections similar to those of Fig. 2. The dark regions illustrate the portion of each die that must be modified to realize a revised die geometry as shown in Fig. 4.
If a solid die is used as illustrated by Fig. 4(a), in some cases it may be possible to recut the existing die if the shape change is purely subtractive and the location is easily accessible, but often the new die geometry must be cut from an entirely new piece of stock to realize the revised geometry. The profile change is less costly when layered dies as shown in Fig. 4(b) and (c) are used because only select layers of each die need to be altered. While saving material, the most important savings are the time and personnel required to effect the modification.
3.5 Potential Limitations
Although there are many potential benefits of using layered extrusion dies, their application is not without limitations. Flexural strength may be limited if unbonded die layers are used. This is particularly relevant for layers near the extrusion orifice. This may place lower bounds on the thickness or material properties selected for die layers. It is critical to calculate the die deflection during extrusion and compensate for it in the die design stage. This deflection can be responsible for a lack of performance in terms of achieving the required geometrical tolerances. Problems with die layer deflection and material ingress in layer-based tooling have been comprehensively discussed in the literature by Soar and Dickens (Ref 9).
Complex dies that produce hollow product by using mandrels may not be well suited for unbonded LMT application. If these techniques are to be used, it may be necessary to employ conventionally manufactured mandrels and bridges, unless it is possible to realize these components using thick layers stacked into the die.
None of these problems appear to be insurmountable or make the further exploration of layered extrusion dies unfavorable.
Fig. 4 Selective die layer substitution example
Fig. 5 Die stack for initial die design Fig. 6 Die stack for improved die design
4. Experimental Results and Discussion
A series of preliminary experiments were carried out to evaluate the merit of the proposed technique. A key blank shape similar in geometry to the work of Ref 18 was used for the extrusion as a compromise between the complex shapes used in industry and the simpler shapes required for basic research. Initial experiments were conducted on plastic dies useful for model material extrusion. Plasticine was used as a modeling material, as the nature of its flow at room temperature is quite similar to that of aluminum at hot extrusion temperatures. This physical modeling technique has been applied successfully to metal flow analysis in various metalforming processes and detailed description of this technique applied to extrusion is given by Prats and Misiolek (Ref 4).
4.1 Die Configurations
The die design was done for the keyhole shape presented in Fig. 5 to 7. The layers were designed as perpendicular edge layers, and all layers were standardized at a thickness of 6.35 mm (0.250 in.) to avoid the complexity of acquiring and managing material of multiple thicknesses.
Two die configurations for the same extruded shape were designed to highlight the utility of the layered extrusion die concept. These are shown in Fig. 5 and 6. Of the nine layers comprising each die, all but three layers are the same in both dies. Consequently, only a total of twelve layers had to be manufactured. The layers that were identical in both designs were shared, and the three different layers were exchanged to form two unique die geometries. Additionally, by removing select layers it was possible to further alter die shape without requiring the creation of any new die hardware. This affords great flexibility and significantly enhances the efficiency of the trial-and-error die development process.
4.2 Die Fabrication and Experimental Setup
Machining of the dies was performed on a relatively inexpensive benchtop NC milling machine. The NC code was generated directly from the CAD drawings via CAM software. Figure 8 shows the assemblage of die layers used for an extrusion trial. Two pins, with a press fit clearance to holes in each die layer, were used to ensure proper alignment between all die layers. A backer plate of 0.500 in. thickness, not shown here, was used to prevent excessive deflection or fracture of the die layer containing the extrusion orifice. This die stack was centered on the press using an annular ring with an insert that mates with the press container. The insert has two holes to accept the die layer alignment pins. This entire assemblage was mounted to the press with a bolster plate and four bolts
4.3 Material Flow
Plasticine with a grid pattern imprinted on a center parting surface of the billet was used for all extrusion trials. After extrusion, the plasticine was removed from the press container, and the die layers were separated one by one from the extrudate remaining in the deforma